Unlocking a new material paradigm
Building the foundation for new advanced materials and high-tech applications of the future
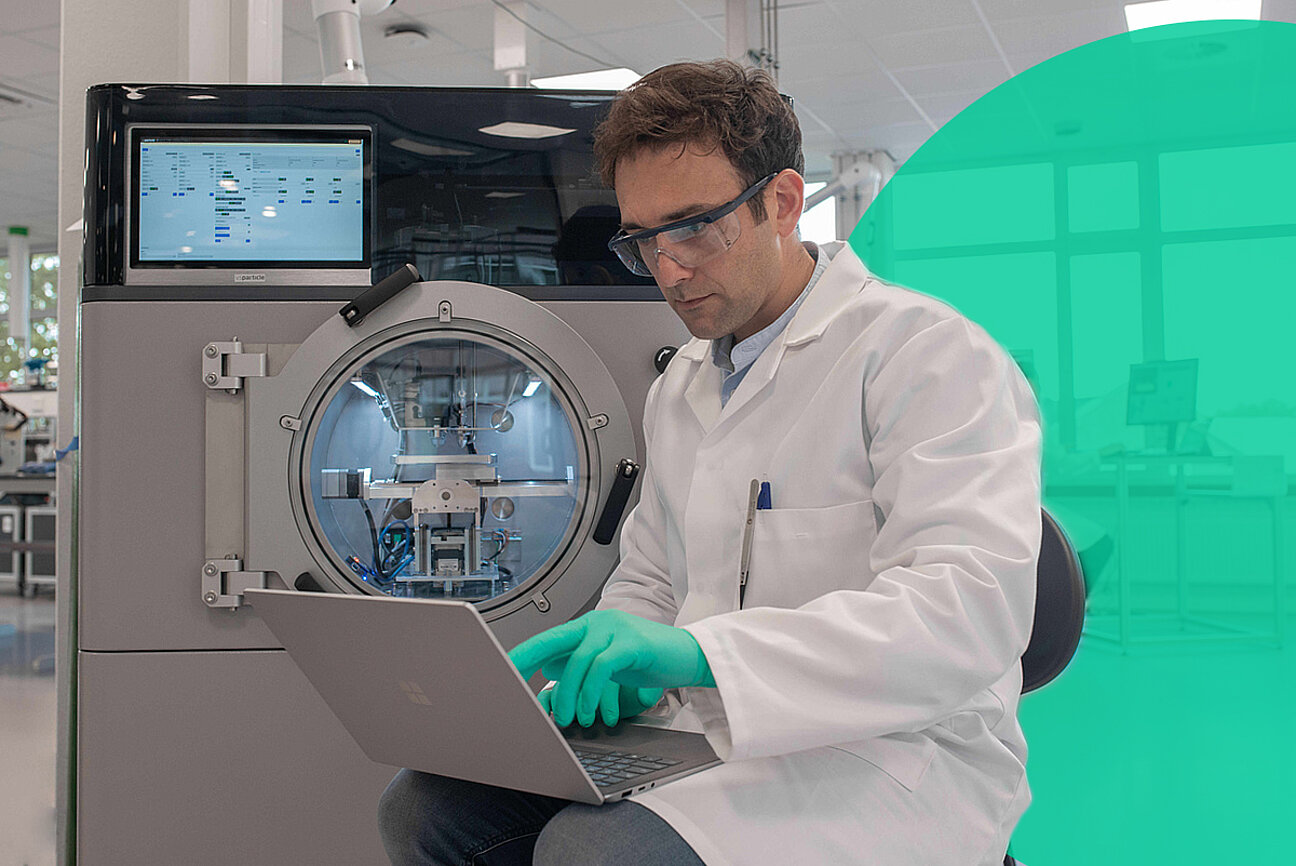
Current way to develop new advanced materials: Mixing bulk materials
Designing and engineering basic materials around us is what has enabled civilisation to become what it is today. Adding value to the materials we mine or grow or collect has proven to be a successful approach in making our lives better.
We even define human evolution based on our use of materials. The stone age, the bronze age, the iron age and more recently the semiconductor age. Every new materials age has provided a new era of possibilities that addressed each societies’ unique challenges.
There are 95 different naturally occurring elements on Earth. The ability to mix these elements enables the production of new advanced materials. By mixing elements together we can optimize physical properties of a material and tailor it to specific needs and applications.
State-of-the-art techniques in materials research has drastically expanded our ability to create more advanced materials. Interestingly, the largest leaps forward in material advancement is direct a result of our ability to produce and manipulate materials at the smallest scale: the nanoscale.
Breaking bulk materials into nanoparticles enables new possibilities to change material properties due to quantum mechanics.
Quantum mechanics changes the properties of a material. When you go from macro to Nano, or from bulk size materials to atom size materials, properties change. Materials start exhibiting unique optical, thermal and electrochemical properties. Nano-scale particles of inorganic materials (nanoparticles) have been proven to be the ideal building blocks to enable next generation batteries, sensors, fuel cells and industrial catalysts.
And this is just the beginning.
The new world of possibilities unlocked by the unique properties of elements at the nanoscale, is expanded by the prospect of unique synergistic effects embodied in novel nanoscale alloys and composites. This new dimension of nanoscale materials places us at the beginning of a new materials revolution in which we are yet to discover 1 % of what is possible.
Combining elements at the nanoscale, manipulating their size and structure, will enable us to prepare materials that were never possible before. While nature has given us an abundance of material potential configurations, society has not had the right tools to explore and create them, until now.
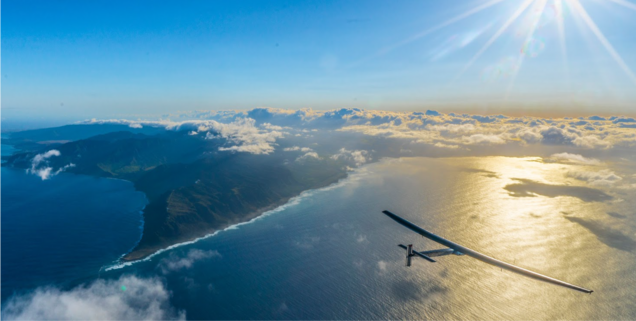
VSPARTICLE has unleashed the full power of nanotechnology and quantum properties by making the production of nanoparticles as easy as pushing a button. By leveraging state of the art automation and machine learning we can fully automate, scale and digitalize material production.
The Nanoparticle source of all VSPARTICLE products
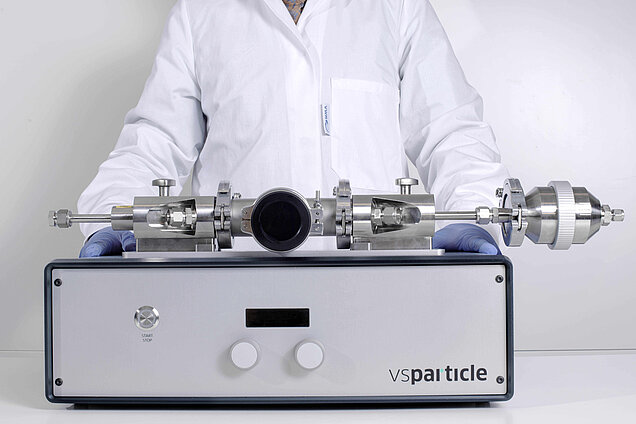
VSPARTICLE goes beyond the current standard in research and introduces a fully automated system that can not only generate the desired nanoparticles but can also print them directly into a new product or integrate the nanoparticles as additional features.
One of the first applications where VSPARTICLE is really introducing the technology for industrial manufacturing is the production of new sensors. The sensing capabilities of nanoparticles and the nano-porous morphology of the printed nanomaterials is very important for such applications.
We are enabling customers to creating new materials with different physical properties and printing them directly in the form of a new product. VSPARTICLE technology is a complete solution and is currently scaled to enable mass manufacturing.
The combination of these two steps in one machine makes the VSP-P1 NanoPrinter a unique technology.
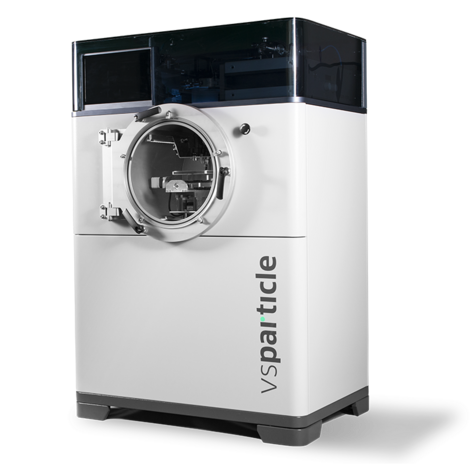
The first validation of the technology came with the release of the smart contact lenses. We started with standard bulk gold rods which we broke into very small gold nanoparticles, by using VSPARTICLE patented Spark Ablation Technology, and directly printed them in the final feature. By using the special quantum properties of gold nanoparticles, we were able to melt the gold at lower temperatures.
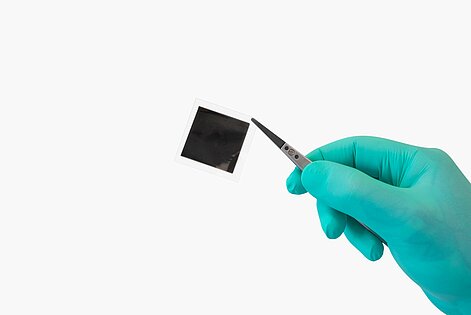
Building the foundation for new advanced materials and high-tech applications of the future
Since VSPARTICLE is enabling a fully digital material creation process is it able to close the scientific loop enabling High-Throughput Material Research in the field of inorganic materials.
Leveraging experimental results with Machine Learning VSPARTICLE can build a bigger VSPARTICLE builds the foundation for new advanced materials and high-tech applications of the future.
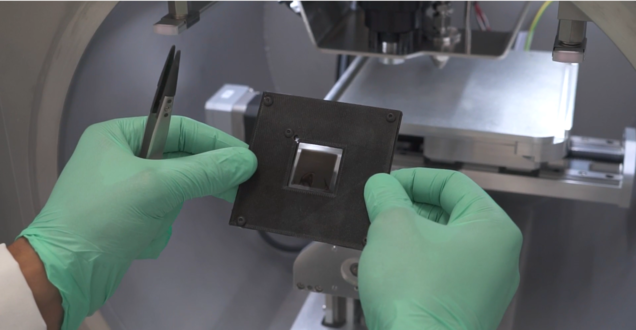