Quantum effects in materials
Many day to day phenomena in all kinds of materials find their origin in quantum mechanics. Conductivity could for example be described by the transport of electrons, colour by the behaviour of photons and magnetism by the interaction of spin states.
On a macroscopic scale the behaviour of a material is usually approximated by a classical description, since most quantum effects are only relevant at the nano scale or at low temperatures, where the behaviour of individual particles and their collective excitations play a bigger role.
Some materials can however be designed such that also on a larger scale, or at higher energies, quantum effects remain significant, giving rise to unique properties that cannot be explained in a classical way.
These so called quantum materials have become a great point of interest for many fields of research and different applications aimed at revolutionizing computing, telecommunication, and consumer electronics.
The physics behind quantum materials
Many quantum materials are developed by reducing their dimensionality, such as graphene, where electrons are confined into a two-dimensional sheet. Furthermore they often accommodate such strong correlations between electrons, that these cannot be described individually but as their collective excitations, known as quasiparticles.
A famous example is the cooper pair: the strongly bound pair of electrons giving rise to superconductivity, currently used to generate the high magnetic fields in MRI scan devices.
The properties that arise in these materials, originating from things like electron confinement and quasiparticle behaviour, will enable ground breaking solutions to many of our modern day problems. These strong interactions for example, leading to collective quasiparticle excitations, redefine our definition of the term current.
In classical physics a current describes the transport of charge, carried from one place to the other by traveling electrons. In quantum materials a current can refer to the transmission of any quantum property or excitation or quasiparticle, providing new and more efficient ways of data storage and transmission or energy conversion.
In a ‘spin current’ for example all electrons stay in the same place and only their spin property is transported from one place to the other, sending a signal through an electrically insulating material without actual moving particles. These new types of current, together with many other unique quantum material properties, are expected to play a big role in future developments in energy harvesting, quantum computing and dissipationless electronics.
The development of new nanomaterials uncovers their quantum properties, making efficient nanoparticle processing the key to developing new quantum materials.
VSPARTICLE has unleashed the full power of nanotechnology and quantum properties by making the production of nanoparticles as easy as pushing a button
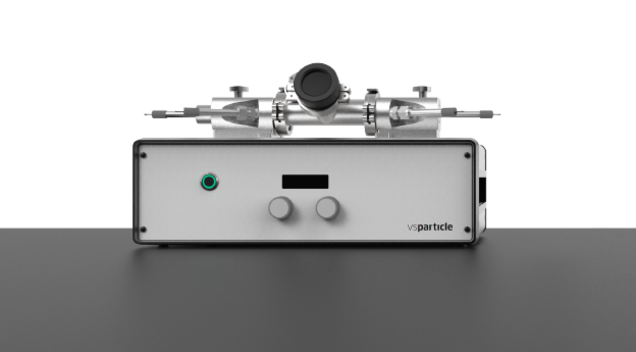
With current methods, such as wet chemical synthesis, it may take months and dozens of iterations to create potentially promising nanomaterials with useful quantum properties.
With VSPARTICLE techniques however, nanoparticles can be created with at the push of a button, easily controlling the size and composition, both speeding up the development of new materials and enabling their scale up for industrial purposes. This unique way of nanoparticle processing makes our technology the perfect tool for the development of quantum materials that will be used in the future generation batteries, fuel cells, solar cells, computers and medical imaging and diagnostic devices.
ENHANCING RAMAN – Sers substrates with unmatched reproducibility and sensitivity
Our first scalable application of quantum materials is already available. With our Surface Enhanced Raman Spectroscopy (SERS) substrates, produced by the VSP-P1 NanoPrinter, Raman signals can be drastically enhanced by several orders of magnitude.
Collective oscillations of electrons in nanostructured metal surfaces, known as Localised Surface Plasmons, are excited by the incoming light beam of the Raman spectrometer, enhancing the local electromagnetic field intensity, and enabling even the detection of single molecules. Applications of this extremely sensitive detection method can be found in fields such as virus detection, drug tests and food safety.
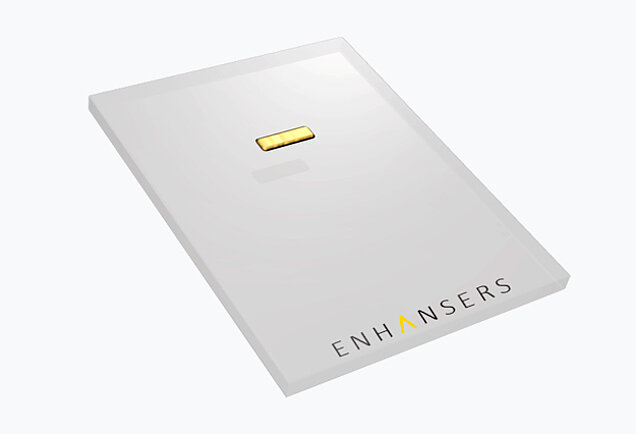
Nano structured materials have proved to be the ideal building blocks to enable the next generation of batteries, sensors, fuel cells and industrial catalysts. And that’s because when you go from macro to Nano, or from bulk size materials to atom size materials, properties change. We provide you with the right tool to explore unique material properties and speed up the development of quantum materials.
Browse through the different sections to learn more about what we can do for your field of interest.