How Nanotech and Catalysis Can Slow Climate Change
Q&A with Wilson A. Smith, associate professor, Department of Chemical Engineering, TU Delft
The Chemical Industry is driven by catalysts, those chemicals that speed up chemical reactions, and with the ability to improve these processes, nanotechnology is set to have a major impact on clean energy development in areas such as hydrogen and liquid fuel production and combustion technologies.
As an associate professor in the Department of Chemical Engineering at TU Delft, Smith has many interests, but he has chosen to turn his focus to slowing down climate change, primarily by enabling energy transition that is centered on renewables. He points out that catalysis is key here because it not only naturally enables so much of every day life on the planet, but also has the potential to address climate change and make life more comfortable, for billions of people around the world, especially those in developing nations.
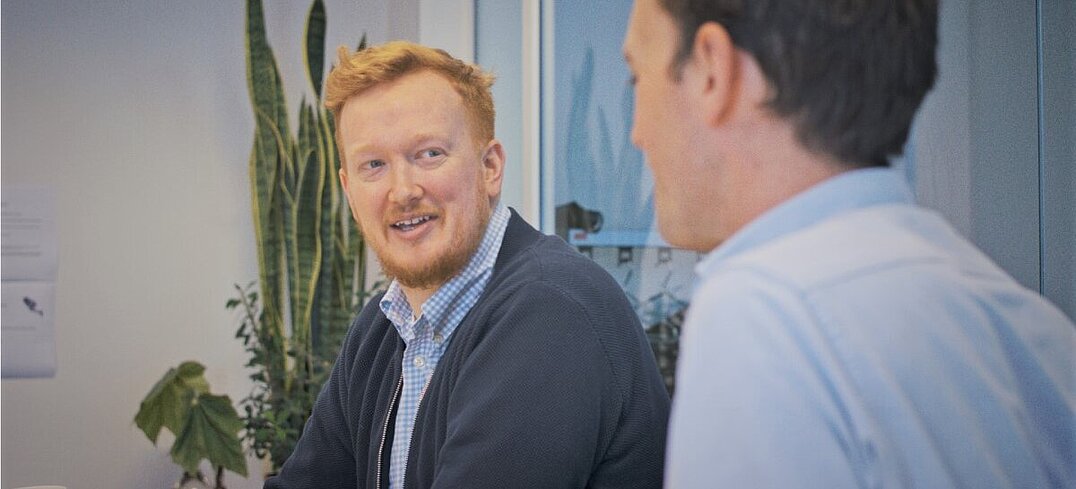
How can catalysis help with reversing climate change?
Catalysis engineering can provide ways to create alternative fuel sources and chemicals to replace fossil ones. One of the main tasks for the coming decades is to leave as much oil, gas, and coal in the ground as possible so we can decrease the amount of greenhouse gases we put into the air. Therefore, finding alternative ways to create the chemicals, fuels, plastics, pharmaceuticals, etc. we still use daily, from non-fossil resources, is imperative to slowing down, and potentially reversing, the catastrophe of climate change. Catalysis is a key technology for making that happen, and, in particular, electrocatalysis offers the potential to use renewable energy from things like solar and wind, to power the process.
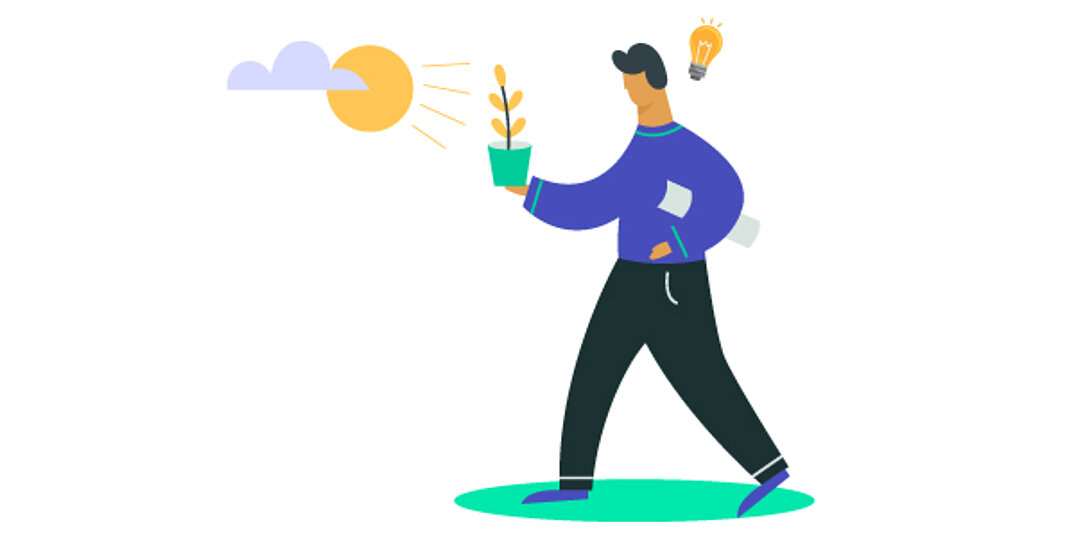
How is Nature, itself, inspiring scientists in this area?
Nature has had a few billion years head start on how to use water, CO2 and sunlight to create complex molecules and fuels. Plants make sugars and oxygen out of water and CO2, and with great energy efficiency, but we struggle to make similar things synthetically and at a reasonable cost. While Nature has the pathway to make complex molecules, it doesn’t need to make many for sustenance, nor does it require much energy because plants are stationary and don't need energy to move around.
Nature has had a few billion years head start on how to use water, CO2 and sunlight to create complex molecules and fuels. Plants make sugars and oxygen out of water and CO2, and with great energy efficiency, but we struggle to make similar things synthetically and at a reasonable cost.
Can you talk more about biomimicry and the research being done into creating an artificial leaf, for example?
Nature does a great job with certain processes, but the scale and thus the energy/fuel needed is different from what we need. As a society, we need, on the order of terawatts (TW) of energy production, Nature’s efficiency is simply too slow. Therefore, instead of mimicking Nature, I think we should take the designs and ideas that work from it, and then use these principles to make something much more robust, faster, and more efficient. For example, in Nature, birds flap their wings to fly. We don't build planes that flap wings, but use jet engines to provide enough lift force to allow us to fly. Here we don't use biomimicry, but the idea that we need vertical thrust to fly, and we accomplish it in a more practical way. Similarly, I don't believe an actual 'artificial leaf' will ever be able to provide the massive amount of chemicals/fuels that we will need, simply because the rate of production in plants is so small compared to what is needed on a global scale.
What are the biggest areas where catalysis can play a major role?
In the current area I am working, electrocatalysis, there are huge opportunities. The technology is still in its relative infancy, so there is plenty of room for fundamental studies, but also opportunities to begin thinking about scalability and translating the lab-scale work to large-scale systems. Here, we should leverage what other heterogeneous catalytic processes have done, so we don't have to re-invent the wheel.
The technology is still in its relative infancy, so there is plenty of room for fundamental studies, but also opportunities to begin thinking about scalability and translating the lab-scale work to large-scale systems.
Are companies working in the area already? What challenges do they face on the road to success?
There are a few startups working on scaling up electrocatalysis, but none are commercial and make products at an economic scale. It is still important for these companies to scale-up so we can learn more about what the challenges are at larger scales, and how this can feed the next generation of basic science inquiry.
Where does nanotech fit into this area?
To make reactions happen at higher rates, we need atomic and nanoscale control over catalysts. Reactors can feed reactants and remove products using mass transport approaches, but the magic happens at the nanoscale. Therefore, even as we scale up these processes to industrial levels, they will all still rely on having nanoscale control.
Where do you see nanotech helping in the future in this area?
In principle nanotech can be used to make better catalysts, as increasing surface area for a reaction (by making things nano) should allow more of the reaction to happen. In addition, for complex reactions that require many steps, being able to control atomic and nanoscale features of a catalyst can allow the ability to tune each step of an overall reaction, making the whole process more efficient in the end.
More about Smith:
Wilson A. Smith is an Associate Professor in the Department of Chemical Engineering at Delft University of Technology. He earned his BS in Physics from American University in 2005, and his Ph.D. in Physics from the University of Georgia in 2010, where he studied the synthesis and applications of nanostructured photocatalysts. From there he moved to Paris, France as a postdoctoral research associate at the Universite Pierre et Marie Curie/Sorbonne where he studied the defect structure of doped semiconductors for solar water purification. In 2012 he began his current position at TU Delft, where his group in the Smith Solar Lab focuses on fundamental processes related to photoelectrochemical water splitting, electrocatalytic water oxidation, and CO2 reduction catalysis using inorganic nanostructured materials.
read more: