Cluster catalysts: unravelling the power of tiny titans │ Introduction to atomic clusters and their application in electrocatalysis
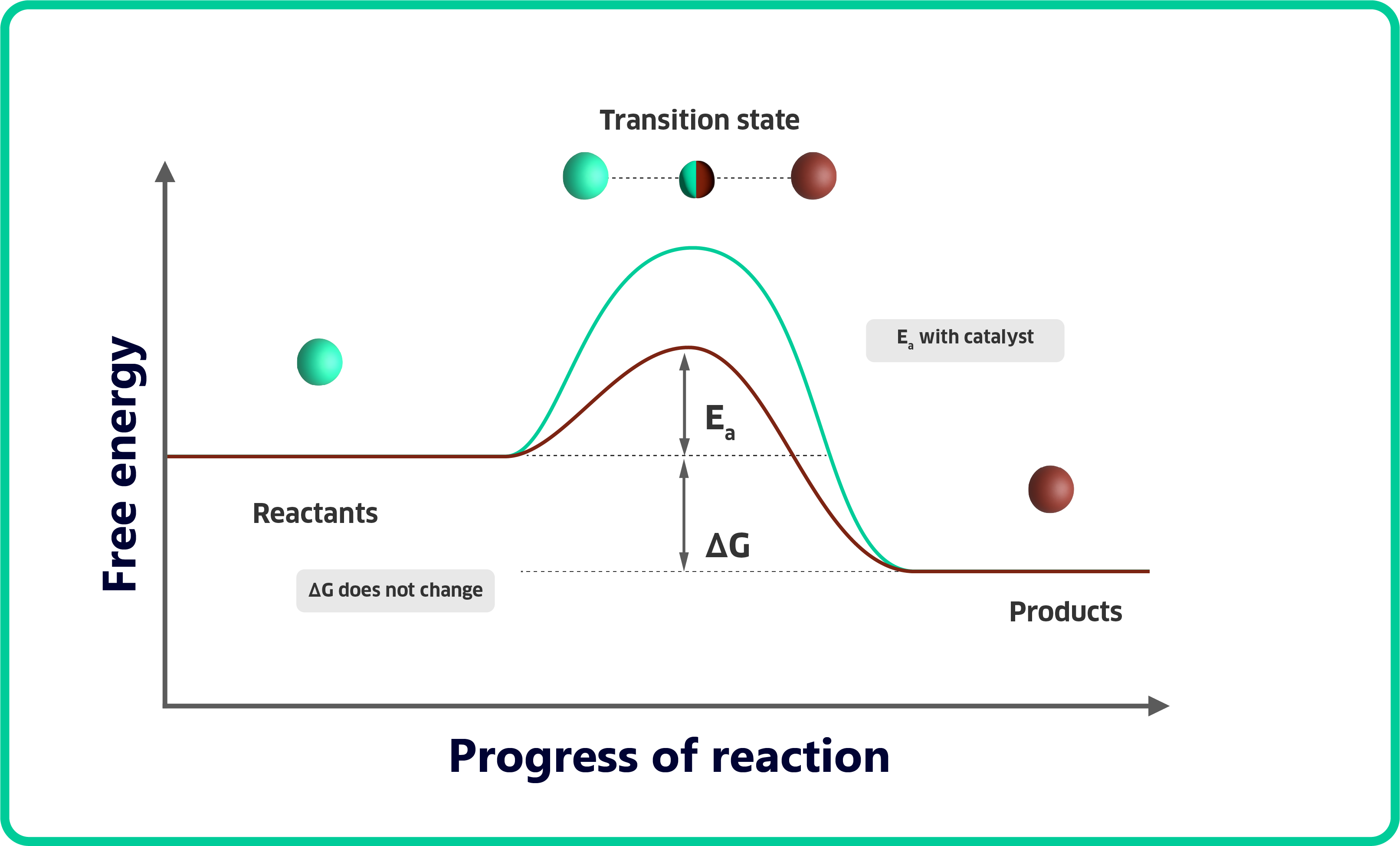
The world of chemistry is often painted with complex equations and abstract concepts. One such concept, catalysis, might leave you wondering: what exactly happens behind the scenes when a catalyst speeds up a reaction? While a textbook might define a catalyst as: "any substance that lowers the activation energy of a reaction," it doesn’t necessarily paint a pretty picture. It hardly captures the intricate dance between reactants and catalysts to guide their transformation into products.
Enter transition state theory, a powerful tool that sheds light on the true magic of catalysts. Imagine a reactant molecule, molecule A, on its journey to become product B. The path isn't always smooth; there's an energetic hurdle it needs to overcome, known as the transition state. The ease at which this hurdle can be overcome determines the rate of the reaction. A catalyst has the ability to change molecule A so that it starts to resemble product B. And this speeds up the reaction!
Catalysts exist all around us: in our bodies, as proteins to help break down toxins or build muscle; in plants, to harvest sunlight and produce sugars; and in the chemical industry to provide us with the materials and technologies we need to shape our world, provide us shelter, keep us safe and healthy, and provide us with the energy needed not just to survive but to thrive.
The dire need for more sustainable catalytic processes

Finding better, cheaper, and more sustainable catalysts has always been at the forefront of science but has never been more pressing. Our ever-increasing energy demands fuelled by fossil fuels have triggered climate change, with CO2 atmospheric levels soaring from 280 parts per million (ppm, unit of concentration) to 400+ ppm and temperatures rising by over 1°C with potential consequences. [1,2,3,4 ]
Further, our oceans are getting more and more polluted, with us dumping 5-10 million tons of everlasting plastics each year (or the equivalent of 20,000 Airbus A380s).[5] How can we create a truly sustainable society?
Nanoparticles our saviours!
Nanoparticles, particles 1000 times smaller than human hair, have found widespread applications as catalysts to help improve the sustainability of our economy. They can have a tremendous capacity to change the configuration of important reactants such as CO2, N2, and H2O. Molecules that are generally relatively inert and hard to change. Those types of reactants are exactly what we would like to start using as a resource and at scale to make our economies more circular.
However, nanoparticles come with a downside. Even though they are 1000 times smaller than human hair, compared to a reactant such as CO2, they are quite big. For a particle of 100 nm, some ~100,000 CO2 molecules can absorb simultaneously. This means that, in effect, one such nanoparticle is actually ~100,000 smaller catalysts at the same time. Sadly, the ability of any of these so-called ‘active sites’ to catalyze the reaction of molecule A to product B is not equal. Often, only a few specific sites on the nanoparticle surface are doing most of the work. The rest of the particle is inert. Or worse yet, it catalyzes a side reaction. Would it not be better to produce a catalyst that only exist of that one specific site? The site that is doing all the work?
Cluster catalysts: tiny titans
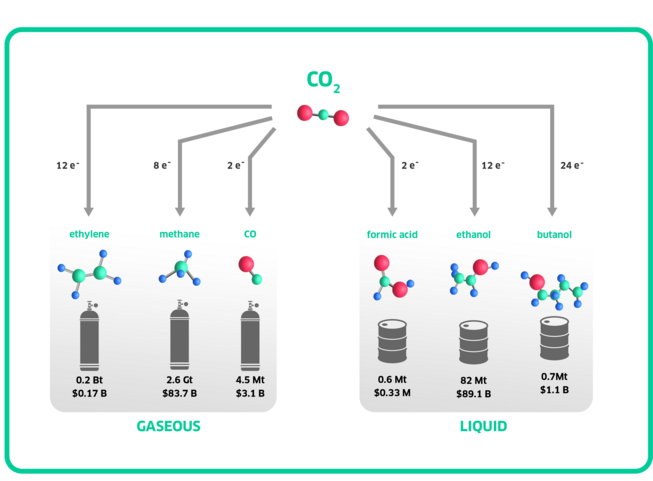
This is exactly what researchers are attempting to do now. Instead of making extremely small but in view of a reactant large nanoparticles, they are producing clusters.[6,7,8] Clusters are groups of atoms bonded together of well-defined atomicity. That is, of a well-defined total number of 100 atoms or less.
Due to their size (<2 nm), clusters can accommodate only a few reactants at a time. Further, their structure and geometry are really uniform. This means that if one finds the right cluster, the cluster that brings reactant A to resemble reactant B closely, one could pack a catalyst surface full of ‘tiny titans’ that are great in converting A to B. [9] And all that former dead space of the inactive part of a nanoparticle is now in good use. This makes the catalyst as a whole much more efficient and much cheaper. As catalysts can get rather expensive, this can make all the difference. For example, Iridium, the go-to catalyst in water splitting, has a 200,000 USD/kg price. Using clusters instead of a 100 nm particle would reduce the cost of the catalyst by a factor of 50!
To address the real challenges of our time—such as minimizing our carbon footprint, producing fertilizer from sustainable sources, breaking down and reusing plastic deposits, and achieving a truly circular economy—we must discover more efficient catalysts. More importantly, once discovered, we should rapidly scale their production to make a significant impact.
Introducing VSParticle’s innovative printing technology
Transitioning into innovative solutions, VSParticle emerges as a trailblazer in the field of nanoparticle generation and printing. The company’s flagship product, the VSP-P1 NanoPrinter with an integrated VSP-G1 Nanoparticle Generator, enables fast and easy synthesis and printing of (semi-) conductive nanoporous layers for various applications.
The versatility of the VSP-G1 Nanoparticle Generator offers researchers the opportunity to reduce the size of particles all the way to the cluster regime and even control their composition. [10]
With the VSP-P1 NanoPrinter, these clusters, by themselves or decorated onto suitable support nanoparticles such as Al2O3, SiO2, TiO2, etc, can be printed on nearly any substrate, i.e., polymers, indium tin oxide (ITO), glass, gold, carbon, etc., directly producing a catalytically active and mechanically stable catalyst layer ready for testing. [11]
VSParticle aims to occupy the space of high-throughput experimentation in which automated closed-loop catalyst optimization schemes are used to accelerate catalyst discovery and optimization vastly. VSP-P1 NanoPrinter and software allow for precise systems control and reproducible results. VSParticle is developing new generations of printer technology to scale catalyst production to industrially relevant capacities.
Acknowledgment
Special thanks to Dr. Cedric David Koolen, Principle Investigator at EPFL, for sharing his knowledge and expertise.
References
(1) Ritchie, H.; Roser, M. Energy. Our World in Data 2020.
(2) US Department of Commerce, N. Global Monitoring Laboratory - Carbon Cycle Greenhouse Gases. gml.noaa.gov/ccgg/trends/ (accessed 2021-08-16).
(3) Stips, A.; Macias, D.; Coughlan, C.; Garcia-Gorriz, E.; Liang, X. S. On the Causal Structure between CO 2 and Global Temperature. Sci Rep 2016, 6 (1), 1–9. doi.org/10.1038/srep21691.
(4) Fourteenth Session of Working Group I and Fifty-Fourth Session of the IPCC — IPCC. www.ipcc.ch/meeting-doc/ipcc-wgi-14-and-ipcc-54/ (accessed 2021-08-16).
(5) Haward, M. Plastic Pollution of the World’s Seas and Oceans as a Contemporary Challenge in Ocean Governance. Nat Commun 2018, 9 (1), 667. doi.org/10.1038/s41467-018-03104-3.
(6) Maisser, A.; Barmpounis, K.; Attoui, M. B.; Biskos, G.; Schmidt-Ott, A. Atomic Cluster Generation with an Atmospheric Pressure Spark Discharge Generator. Aerosol Science and Technology 2015, 49 (10), 886–894. doi.org/10.1080/02786826.2015.1080812.
(7) Maisser, A.; Barmpounis, K.; Holm, S.; Attoui, M.; Schmidt-Ott, A.; Kangasluoma, J.; Biskos, G. Characterization of Atmospheric-Pressure Spark Generated Atomic Silver and Gold Clusters by Time-of-Flight Mass Spectrometry. Journal of Aerosol Science 2021, 156, 105780. doi.org/10.1016/j.jaerosci.2021.105780.
(8) Koolen, C. D.; Luo, W.; Züttel, A. From Single Crystal to Single Atom Catalysts: Structural Factors Influencing the Performance of Metal Catalysts for CO2 Electroreduction. ACS Catal. 2022, 948–973. doi.org/10.1021/acscatal.2c03842.
(9) Arenz, M.; Gilb, S.; Heiz, U. Chapter 1 Size Effects in the Chemistry of Small Clusters. In The Chemical Physics of Solid Surfaces; Woodruff, D. P., Ed.; Atomic Clusters: From Gas Phase to Deposited; Elsevier, 2007; Vol. 12, pp 1–51. doi.org/10.1016/S1571-0785(07)12001-0.
(10) Scalable synthesis of Cu(-Ag) oxide clusters via spark ablation for the highly selective electrochemical conversion of CO2 to acetaldehyde. doi.org/10.21203/rs.3.rs-3791391/v1.
(11) http://dx.doi.org/10.1016/j.apt.2013.12.005
-----------------
Comments
The publication: ‘Scalable synthesis of Cu(-Ag) oxide clusters via spark ablation for the highly selective electrochemical conversion of CO2 to acetaldehyde,’ is a preprint and has not yet undergone peer review. Therefore, its findings are provisional and its conclusions may change.
Comments
No Comments