Nanoporous marvels | Introduction to nanoporous materials and their application in electrocatalysis and gas sensing technologies
Nanoporous materials or layers refer to substances with structures characterized by a network of pores or voids at the nanometer scale, typically ranging from 1 to 100 nm in size. The nanoporous nature of these materials provides an exceptionally high surface area relative to their volume, which is a key factor in their utility. This heightened surface area allows for increased interactions with other substances, such as gases, liquids, or molecules, making nanoporous materials valuable in a range of applications.
According to the International Union of Pure and Applied Chemistry (IUPAC), nanoporous materials can also be classified based on pore size: microporous material (pore sizes from 0 to 2 nm, mesoporous material (2 to 50 nm), and macroporous materials (greater than 50 nm). These nanoscale pores endow the materials with unique properties and functionalities, making them versatile for various applications across scientific, technological, and industrial domains.
Nanoporous materials can be found in various forms, including films, membranes, powders, and bulk structures. They can be engineered to have specific pore sizes, shapes, and distributions, allowing for tailoring of their properties to suit desired applications. The ability to manipulate and engineer nanoporous materials opens up exciting avenues for innovation, enabling advancements in diverse scientific disciplines and technological applications.
A plethora of application areas
Over the last decade, there has been an ever-increasing interest and research effort in the synthesis, characterization, functionalization, and design of nanoporous materials. Research efforts in this field have been driven by the rapidly growing emerging applications such as biosensors, drug delivery, gas separation, energy storage, fuel cell technology, and catalysis. These applications offer exciting new opportunities for scientists to develop new techniques for the synthesis and applications of these materials.
Nanoporous materials demonstrate remarkable versatility with impactful applications, notably in the realms of green hydrogen production and gas sensing.
The pressing need for sustainable energy solutions
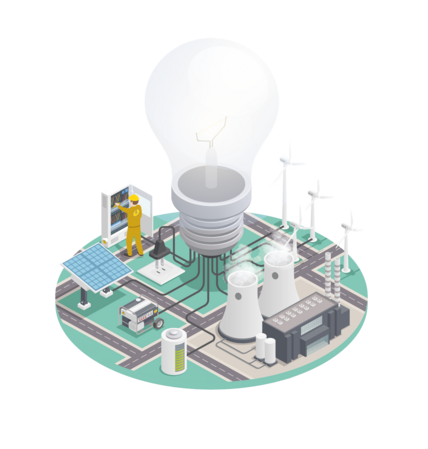
Energy is at the heart of the climate challenge. Fossil-fuel dominated electricity generation in the United States and China has enormous environmental consequences. In 2007, 2.4 billion metric tons of carbon dioxide (CO2) were emitted from electricity generation in the United States, about 40 percent of the country’s energy-related greenhouse gas (GHG) emissions. In the same year, electricity generation in China produced just over 2 billion metric tons of CO2, accounting for about one-third of its energy-related GHG emissions. [1]
This scenario underscores the pressing need to reassess and transition from conventional energy sources to more sustainable alternatives. In this context, the concept of green hydrogen emerges as a promising solution to address the environmental impact of energy production.
Nanoporous materials demonstrate remarkable versatility with impactful applications, especially in the field of green hydrogen production. As a matter of fact, many literature studies already indicate that nanoporous materials offer promising performances in catalyzing and optimizing electrocatalytic processes (such as water electrolysis, CO2 reduction to hydrocarbons etc., etc.) due to their enhanced surface area/ surface energy.
Although the physical and chemical properties in the nano-scale sizes are still largely unexplored, it is already widely assumed that nanoporous materials could be advantageous to improve the thermodynamics of electrochemical reactions at the electrode/electrolyte interface, as well as to promote a better electron transfer. Moreover, the enhanced surface area exposed during an electrochemical reaction provides more active sites and higher mass diffusion capability, which could definitely have a positive impact on its electrochemical performances.
The significance of nanoporous materials lies in their ability to act as highly active electrocatalysts. The performance of heterogeneous catalysts strictly depends on their structures and physicochemical properties. Heterogeneous catalysts with large specific surface areas possess more catalytic sites to enhance the overall catalytic performance. Nanoporous materials emerged as highly active heterogeneous catalysts due to their large internal surface area, enabling a high density of active catalytic sites. The presence of nanopores also allows the selectivity towards the desired products.[2]
The ability of nanoporous materials to enhance catalytic efficiency hugely contributes to the advancement of sustainable and eco-friendly green hydrogen production, a crucial component in the global shift towards renewable energy.
Nanoporous materials in gas sensing technologies
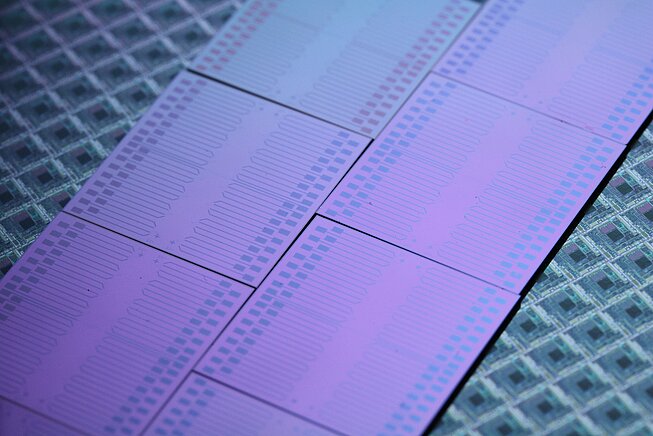
Gas sensing technologies are indispensable for safety, environmental monitoring, and industrial optimization. These sensors contribute to workplace safety by detecting hazardous gas concentrations, ensuring air quality through pollution monitoring, and enhancing industrial processes by optimizing combustion and controlling production. They play a vital role in early fire detection, support energy efficiency efforts by minimizing waste, find applications in medical diagnostics, aid environmental research, and are increasingly integrated into smart home systems for enhanced safety.
In 2020, household air pollution led to approximately 3.2 million deaths, with more than 237,000 fatalities among children under 5 years old. The combined impact of both ambient and household air pollution contributes to around 6.7 million premature deaths each year. Urgent measures, such as the widespread adoption of clean fuels and technologies, are necessary to mitigate household air pollution and safeguard public health. [3]
The current gas sensing technologies are mainly governed by metal-oxides (MOXs) based gas sensors as a result of their gas sensing performances and fabrication methods. For instance, these materials have high sensitivity towards a wide range of gases, fast response and recovery time, high thermal and mechanical stabilities, are easy to miniaturize, and there are relatively low costs involved in their production [4].
However, the major drawback of this sensor technology is its poor selectivity towards certain target analytes [5]. The selectivity of a gas sensor is a paramount performance metric of a sensing device, basically related to the ability to detect a target gas in the presence of other gases. The poor selectivity dramatically affects the reliability of the sensor because the device cannot accurately detect the presence of a specific gas concentration due to its cross-sensitivity with other gases surrounding the sensing device.
In addition to selectivity constraints, the next generation of gas sensors will need to fulfill new energetic requirements to excel in the future Internet of Things (IoT) era. The MOX-based gas sensors are considered to have a low power consumption compared with other commercially available sensors [6]. Nevertheless, the fact that their operation temperature is at least 200 °C can affect the power consumption budget given the massive network of sensors that will need to continuously acquire, analyze and share data [7].
In recent decades, many efforts have been made to outperform the current gas-sensing technology. Nanoporous materials are attractive solutions due to their tuneable structural, thermal, optical, and electronic properties. Besides, their large surface-to-volume ratio significantly increases the active sites interacting with a target analyte, compared with bulk counterparts' sensors. The nanoporous layers can be engineered in many ways, such as creating heterostructures, junctions, and doping the material. Collectively, these properties render the nanoporous materials an essential building block for the conception of the next generation of gas sensors that can reduce the challenges associated with their performance, fabrication, and implementation.
The imperative of developing accurate nanoporous materials
Research and development on technologies to create accurate nanoporous layers are vital for unlocking the full potential of these materials across a spectrum of applications. From catalysis to gas sensing and energy storage, the tailored engineering of nanoporous layers is essential for realizing advanced functionalities that contribute to sustainable and innovative technological solutions. The ongoing pursuit of these technologies is key to addressing pressing global challenges and driving the transition towards a cleaner and more efficient future.
Introducing VSParticle's innovative nano printing technology
VSParticle is a pioneer in the field of nanoparticle generation and printing. The company’s flagship product, VSP-P1, enables a fast and easy synthesis and printing of (semi-) conductive nanoporous layers for various applications.
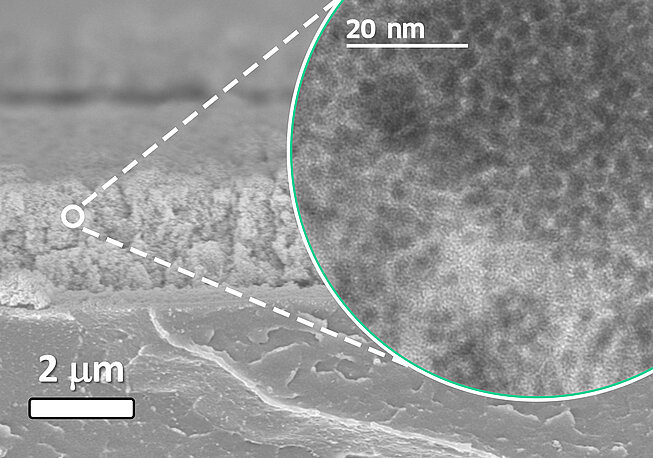
The versatility of the nanoprinters developed by VSParticle is also another aspect that can be exploited in the catalyst manufacturing process. In fact, when the VSP-P1 nanoprinter is coupled with multiple nanoparticles G1 generators, many unexplored materials can be created in a relatively short time, for example, high entropy materials (HEM) with five or more metals in equiatomic or near atomic ratios. Recent studies also showed that many of these materials possess outstanding properties due to (I) a high number of active sites, (II) elemental diversity, (III) optimal interaction between catalyst surface and reaction intermediates. In light of these properties, HEM have also been utilized for water electrolysis, showing superior activity and stability performances with respect to the conventional low-entropy materials.
VSParticle also aims to occupy the field of high-throughput experimentation (HTE), namely a technique that aims to test simultaneously a large number of experiments in a relatively short time. In fact, one of the biggest challenges in electrocatalysis is the exploration of novel electrocatalysts, wherein the nano printer developed by VSParticle is expected to have a great impact on science. The capability to produce a diverse range of nanoporous materials with high reproducibility represents the promise to further boost catalyst discovery in many fields of electrocatalysis, from water electrolysis to oxygen evolution reaction. This will be beneficial for the entire scientific community in order to enrich the existing catalyst libraries and improve the current state—of—the—art of many other electrochemical reactions in a relatively short time.
For more information about VSParticle’s technology and explore the possibilities for your field, please get in touch with us.
Acknowledgment
Special thanks to Leandro Sacco, Application Specialist, VSParticle, and Simone Asperti, Development Engineer Electrocatalysis, VSParticle, for contributing and sharing their knowledge and expertise in writing this blog post.
References
[1] National Academies of Sciences, Engineering, and Medicine. 2010. The Power of Renewables: Opportunities and Challenges for China and the United States. Washington, DC: The National Academies Press. https://doi.org/10.17226/12987.
[2] Kadja, G. T., Ilmi, M. M., Azhari, N. J., Khalil, M., Fajar, A. T., Makertihartha, I., Gunawan, M. L., Rasrendra, C. B., & Wenten, I. (2022). Recent advances in the nanoporous catalysts for the generation of renewable fuels. Journal of Materials Research and Technology, 17, 3277-3336. https://doi.org/10.1016/j.jmrt.2022.02.033
[3] Household air pollution (who.int)
[4] Kim, K., Park, J. K., Lee, J., Kwon, Y. J., Choi, H., Yang, S. M., ... & Jeong, Y. K. (2022). Synergistic approach to simultaneously improve response and humidity-independence of metal-oxide gas sensors. Journal of Hazardous Materials, 424, 127524.
[5] Li, Q., Zeng, W., & Li, Y. (2022). Metal oxide gas sensors for detecting NO2 in industrial exhaust gas: Recent developments. Sensors and Actuators B: Chemical, 359, 131579.
[6] Cho, I., Sim, Y. C., Cho, M., Cho, Y. H., & Park, I. (2020). Monolithic micro light-emitting diode/metal oxide nanowire gas sensor with microwatt-level power consumption. ACS sensors, 5(2), 563-570.
[7] Potyrailo, R. A. (2016). Multivariable sensors for ubiquitous monitoring of gases in the era of internet of things and industrial internet. Chemical reviews, 116(19), 11877-11923
Comments
No Comments